CAZypedia needs your help!
We have many unassigned pages in need of Authors and Responsible Curators. See a page that's out-of-date and just needs a touch-up? - You are also welcome to become a CAZypedian. Here's how.
Scientists at all career stages, including students, are welcome to contribute.
Learn more about CAZypedia's misson here and in this article.
Totally new to the CAZy classification? Read this first.
Auxiliary Activity Family 11
This page has been approved by the Responsible Curator as essentially complete. CAZypedia is a living document, so further improvement of this page is still possible. If you would like to suggest an addition or correction, please contact the page's Responsible Curator directly by e-mail.
Auxiliary Activity Family 11 | |
Clan | Structurally related to AA9 & AA10 |
Mechanism | lytic oxidase |
Active site residues | mononuclear copper ion |
CAZy DB link | |
http://www.cazy.org/AA11.html |
Substrate specificities
The AA11 family of Lytic Polysaccharide Mono-oxygenases (LPMOs; first described in [1]) was identified using a bioinformatic 'module walking' approach [2]. Many AA9 proteins have C-terminal domains, which are often cellulose binding CBMs (eg. CBM1), but some have no known function (also see [3]). Sequence searches using one of these domains (termed X278) returned hits where the domain was fused with GH18 family members and another set of domains of unknown function. Closer examination of these domains revealed that they had a likely signal peptide followed by a histidine, typical of LPMOs, though they otherwise lacked significant sequence similarity to either AA9 or AA10 family members.
The only AA11 to be isolated to to date is from Aspergillus oryzae which showed copper dependent oxidase activity on chitin in the presence of ascorbate. The observed products were a mixture of mainly aldonic acids and unmodified oligosaccharides with a small amount of a -2Da species, which may be C4 oxidation or the C1 lactone prior to ring opening. It is unknown at this stage whether AA11 family members will show any activity towards other substrates, whether they oxidise at positions other than C1 on the sugar ring or what the role of the X278 domain often found at the C-terminus is (although a chitin binding function would seem logical given its presence on GH18 chitinases and AA11).
Kinetics and Mechanism
AA11s, like AA9s and AA10s, are copper dependent mono-oxygenases but the chemical mechanism by which these enzymes perform the reaction is yet to be elucidated. Recent quantum mechanical simulations suggest that AA9s are likely to oxidise cellulose using a copper-oxyl, oxygen rebound mechanism [4] but further work is needed in this area. The differences in the copper coordination geometries between the families might further hint that they might use different mechanisms (Reviewed in [5]). As is the case for AA10s, the natural electron donor for AA11s is unknown, with no equivalent of cellobiose dehydrogenase yet discovered that is active on chitobiose. It is also unknown whether the N-terminal histidine in AA11s is N-methylated (as the enzyme in the published study was expressed in E. coli and not a filamentous fungi) as is seen in AA9s and what effect this will have on the reaction performed by the enzyme.
Catalytic Residues
Like AA9 and AA10, AA11 utilises copper, which it binds through the "histidine brace" consisting of the N-terminal histidine's amino and imidazole groups together with the imidazole sidechain of another histidine. It is unknown at this stage whether AA11s possess the methylated N-terminal histidine observed in AA9s as the protein was produced recombinantly in E. coli which does not carry out this modification. Like AA9, a tyrosine sidechain is in close proximity to the copper ion but it does not directly coordinate the metal in this case. AA9s typically leave the opposite side to the tyrosine open to solvent where water molecules are observed coordinating the metal ion [6, 7, 8, 9]. In AA10s there is an alanine in this position, which causes a distortion in the copper coordination geometry away from octahedral [10, 11, 12, 13]. Interestingly AA11 has an alanine in a very similar position giving an active site structure somewhere between that of AA9s and AA10s. The structure was only determined with Cu(I) in the active site so the coordination geometry of Cu(II) was not directly observed but electron paramagnetic resonance spectroscopy confirmed that the copper coordination geometry lies somewhere between that of AA9s and AA10s [2].
Three-dimensional structures
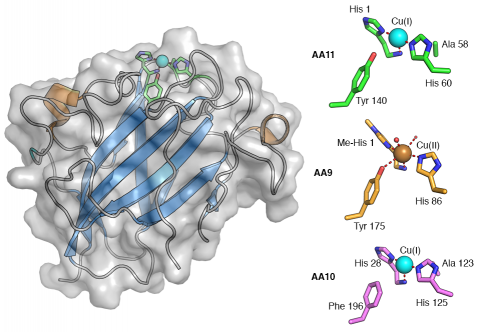
Though AA11 does not share significant sequence similarity to AA9 and AA10 proteins, the core fold of the protein is remarkably similar forming a typical ß-sandwich immunoglobulin like fold [2]. The active site is at the centre of a slightly concave surface which, consistent with observations in AA10s [11, 13], has very few aromatic residues suggesting that it also primarily interacts with chitin via H-bonding interactions. Given that LPMOs are oxidoreductases there is increasing interest in the role of electron transport chains within these proteins [8]. Internal tyrosine and tryptophan residues have been implicated in these roles in AA9s [8] and AA10s [5] respectively. Similarly in AA11s it is possible to trace a path through the core of the protein using tryptophans, methionine and other hydrophilic residues to the distal face of the enzyme from the active site suggesting that there may be a similar electron transport path in AA11s though these are yet to be experimentally verified in LPMOs.
Family Firsts
- First family member identified
- AA11 from A. oryzae [2].
- First demonstration of oxidative cleavage
- AoAA11 was shown to oxidatively cleave squid pen chitin producing a mixture of aldonic acids, unmodified oligosaccharides and undistinguishable lactone/C4 oxidation products [2].
- First 3-D structure
- AA11 from A. oryzae with Zn2+ 4MAH & Cu(I) 4MAI [2]
References
- Vaaje-Kolstad G, Westereng B, Horn SJ, Liu Z, Zhai H, Sørlie M, and Eijsink VG. (2010). An oxidative enzyme boosting the enzymatic conversion of recalcitrant polysaccharides. Science. 2010;330(6001):219-22. DOI:10.1126/science.1192231 |
- Hemsworth GR, Henrissat B, Davies GJ, and Walton PH. (2014). Discovery and characterization of a new family of lytic polysaccharide monooxygenases. Nat Chem Biol. 2014;10(2):122-6. DOI:10.1038/nchembio.1417 |
- Horn SJ, Vaaje-Kolstad G, Westereng B, and Eijsink VG. (2012). Novel enzymes for the degradation of cellulose. Biotechnol Biofuels. 2012;5(1):45. DOI:10.1186/1754-6834-5-45 |
- Kim S, Ståhlberg J, Sandgren M, Paton RS, and Beckham GT. (2014). Quantum mechanical calculations suggest that lytic polysaccharide monooxygenases use a copper-oxyl, oxygen-rebound mechanism. Proc Natl Acad Sci U S A. 2014;111(1):149-54. DOI:10.1073/pnas.1316609111 |
- Hemsworth GR, Davies GJ, and Walton PH. (2013). Recent insights into copper-containing lytic polysaccharide mono-oxygenases. Curr Opin Struct Biol. 2013;23(5):660-8. DOI:10.1016/j.sbi.2013.05.006 |
- Harris PV, Welner D, McFarland KC, Re E, Navarro Poulsen JC, Brown K, Salbo R, Ding H, Vlasenko E, Merino S, Xu F, Cherry J, Larsen S, and Lo Leggio L. (2010). Stimulation of lignocellulosic biomass hydrolysis by proteins of glycoside hydrolase family 61: structure and function of a large, enigmatic family. Biochemistry. 2010;49(15):3305-16. DOI:10.1021/bi100009p |
- Karkehabadi S, Hansson H, Kim S, Piens K, Mitchinson C, and Sandgren M. (2008). The first structure of a glycoside hydrolase family 61 member, Cel61B from Hypocrea jecorina, at 1.6 A resolution. J Mol Biol. 2008;383(1):144-54. DOI:10.1016/j.jmb.2008.08.016 |
- Li X, Beeson WT 4th, Phillips CM, Marletta MA, and Cate JH. (2012). Structural basis for substrate targeting and catalysis by fungal polysaccharide monooxygenases. Structure. 2012;20(6):1051-61. DOI:10.1016/j.str.2012.04.002 |
- Quinlan RJ, Sweeney MD, Lo Leggio L, Otten H, Poulsen JC, Johansen KS, Krogh KB, Jørgensen CI, Tovborg M, Anthonsen A, Tryfona T, Walter CP, Dupree P, Xu F, Davies GJ, and Walton PH. (2011). Insights into the oxidative degradation of cellulose by a copper metalloenzyme that exploits biomass components. Proc Natl Acad Sci U S A. 2011;108(37):15079-84. DOI:10.1073/pnas.1105776108 |
- Hemsworth GR, Taylor EJ, Kim RQ, Gregory RC, Lewis SJ, Turkenburg JP, Parkin A, Davies GJ, and Walton PH. (2013). The copper active site of CBM33 polysaccharide oxygenases. J Am Chem Soc. 2013;135(16):6069-77. DOI:10.1021/ja402106e |
- Vaaje-Kolstad G, Bøhle LA, Gåseidnes S, Dalhus B, Bjørås M, Mathiesen G, and Eijsink VG. (2012). Characterization of the chitinolytic machinery of Enterococcus faecalis V583 and high-resolution structure of its oxidative CBM33 enzyme. J Mol Biol. 2012;416(2):239-54. DOI:10.1016/j.jmb.2011.12.033 |
- Vaaje-Kolstad G, Houston DR, Riemen AH, Eijsink VG, and van Aalten DM. (2005). Crystal structure and binding properties of the Serratia marcescens chitin-binding protein CBP21. J Biol Chem. 2005;280(12):11313-9. DOI:10.1074/jbc.M407175200 |
- Aachmann FL, Sørlie M, Skjåk-Bræk G, Eijsink VG, and Vaaje-Kolstad G. (2012). NMR structure of a lytic polysaccharide monooxygenase provides insight into copper binding, protein dynamics, and substrate interactions. Proc Natl Acad Sci U S A. 2012;109(46):18779-84. DOI:10.1073/pnas.1208822109 |